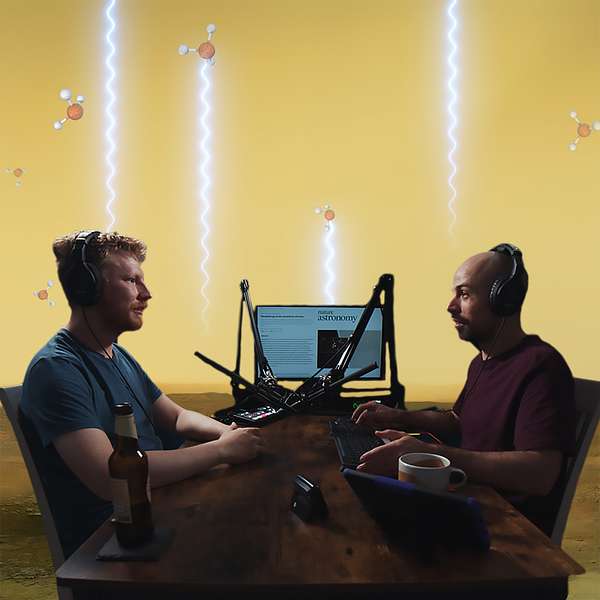
Bullaki Science Podcast
Bullaki Science Podcast
2. Life on Venus? | Dr Paul B. Rimmer
Dr Paul B. Rimmer is one of the scientists who contributed to the discovery of a potential biomarker (phosphine) in the atmosphere of Venus. In this podcast we explore what does this finding actually mean and go through the details of their publication ‘Phosphine gas in the cloud decks of Venus’.
The video podcast is available here: https://youtu.be/syQmBJkpfw8
Paul details the journey of this discovery lead by Prof. Jane S. Greaves, which took almost 4 years and involved a large collaboration including Cardiff University, the University of Manchester, the MIT, Kyoto Sangyo University, the Imperial College, the Open University, and the East Asian Observatory. This publication was authored by Jane S. Greaves, Anita M. S. Richards, William Bains, Paul B. Rimmer, Hideo Sagawa, David L. Clements, Sara Seager, Janusz J. Petkowski, Clara Sousa-Silva, Sukrit Ranjan, Emily Drabek-Maunder, Helen J. Fraser, Annabel Cartwright, Ingo Mueller-Wodarg, Zhuchang Zhan, Per Friberg, Iain Coulson, E’lisa Lee and Jim Hoge.
Paul explains that we do not know any abiotic phosphine (PH3) production routes in Venus’s atmosphere, clouds, surface and subsurface, or from lightning, volcanic or meteoritic delivery. Paul explores the possibility that phosphine (PH3) could either originate from unknown photochemistry or geochemistry, or, building upon a previous work by one of the co-authors of this work (Clara Sousa-Silva), from the presence of life. Finally Paul lays down a roadmap for future investigations to better understand the origin of phosphine on Venus involving a tandem work between laboratory measurements and ground-based telescope observations.
We also discuss the role of media and click bait news, which came out with titles suggesting life on Venus and extraterrestrial or alien life on Venus.
Samuele Lilliu (SL). Paul thank you very much for doing this. You’re all over the news now with this paper Phosphine Gas in the Clouds Decks of Venus.[1] People are saying “maybe there is life on Venus!” What’s the story? Can you give me a brief overview of this paper?
Paul B. Rimmer (PBR). Sure so the main thing that’s been found on the paper is that a particular spectroscopic feature was found in the atmosphere of Venus around 1 mm wavelength and it was found with two different instruments the James Clerk Maxwell Telescope and the Atacama Large Millimeter Array (ALMA). This was observed by Jane Greaves and the only explanation that we’ve been able to find for this feature, of all the known molecules with known features around there, the only one that explains this feature, is phosphine. Phosphine is a molecule composed of one phosphorus atom and three hydrogen atoms. That’s the one. And by all rights…
SL. Let’s uh specify that the molecule doesn’t look like that…
PBR. Oh, yeah so although I’ve been really interested in when people do these sorts of microscopy studies and they see what molecules like benzene rings [2] look like they look surprisingly like the ball and stick models actually. I will admit it doesn’t look exactly like that but it would certainly have a sort of electron cloud in a certain area and three other electron clouds and there’d be a little bit of space between them and if you looked at this with the right kind of microscopy it would look surprisingly like that picture.
SL. Okay, so we’ve seen all this news, sort of click bait news. This one for example Signs of alien life detected on Venus or this other one Forget Mars: scientists find a sign of life on Venus. What do you think about this click bait news?
PBR. I definitely understand why it exists because people want to make money and this is a good way to get people to click. Like you say, it is click bait. I think that it does have something in common with fake political news in terms of saying something outlandish so that people will click so that money is gained. The particular algorithms happen to be satisfied so that more people see a particular news articles. I do think that it’s damaging to science, I think it’s damaging to the search for truth in general though actually. I think that it’s important that scientific papers report what they see. It’s fine to speculate if there’s a basis for that speculation, but it’s important to be clear about what the evidence actually is, what the speculation is, and how those two are related, and what predictions can be made to really test these speculations.
SL. The thing is that, if a scientist sees this, [the scientist] will probably understand that there is some exaggeration. That’s probably a title just to grab the attention. But if you take an average person, maybe [that person] might think “well actually scientists found life on Venus or on Mars!”. If you remember, I think there was something about methane and Mars and people were speculating that that [methane] could be a biomarker.
PBR. Yeah, I’ve done some research on methane on Mars as well actually.[3] There are alternative ways to make methane. Renyu Hu has a wonderful paper where he comes up with five different ways only one of which happens to be life.[4] This is a very similar sort of story, except that it’s earlier on. Right now there is no known explanation for the phosphine [on Venus] besides life that would produce it in the quantities needed. But that’s just because there’s a lot less that’s known about phosphine than methane. I would imagine that there will be alternatives at some point in time once people really have the chance to seriously study it in this kind of environment.
SL. So there are actually more people studying methane than phosphine…
PBR. Oh yes…
SL. and for obvious reasons, right?
PBR. Yes, methane is more prevalent. It’s been considered a biosignature for a lot longer and it’s a lot safer to work with. Phosphine is incredibly lethal and it’s explosive. It’s not a fun thing to work with.
SL. There are also positive aspects in this clickbait news because if you do this sort of news, if the news spreads, then that might reach policy makers, politicians, and that can help with the allocation of funds for this specific research, maybe it can reach some philanthropist.
PBR. Oh yeah. So the way that I would respond to that is if the news is reported honestly then I think that that can be very useful. If the news is reported dishonestly I think it can be kind of like a poison apple, in the sense of if you say that you find life somewhere or that you could find life somewhere and then you finally check and you’re not careful and you don’t find it, it feels very much like scientists are lying. This, I think, is damaging in two ways. One is it’s going to get rid of further funding to search for interesting things on Venus or wherever else you happen to be searching. But it’s also damaging because there’s really well-established science, science that’s not at the beginning of the story but established further along in the story. Like climate science. So people say “well, look, scientists don’t know what they’re talking about with phosphine on Venus, scientists don’t know what they’re talking about with global climate change”. You don’t want to equate those two and there’s a risk that that happens when people say such confident and definitive things in titles of articles and this is especially damaging if politicians or philanthropists happen to read this, happen to believe it, and then happen to be disillusioned by it.
SL. Hopefully we won’t get the same sort of polarization… you’re going to get one party that is going to be for Mars and the other one for Venus… the same way it happens with climate change.
PBR. No… I certainly hope that that doesn’t happen. Ideally both should be pursued because both can answer very different questions. There are some really important questions that can only be answered on Mars that just cannot be answered on Venus and vice versa.
SL. Yeah it’s very different… it’s a very different atmosphere, right?
PBR. It’s a different atmosphere and it’s a different surface. We don’t know as much about the surface of Venus because it’s so hostile. But there is some evidence that it was resurfaced relatively recently, meaning only in the last 600 million years [5]. Very important to understand how volcanic or magmatic processes might work, but very frustrating if you want to find out what early Venus was like. It’s all been erased. Whereas with Mars, since it doesn’t have the tectonic activity you can go right back to almost this formation.
SL. So that’s probably what’s the impression that most people have about this this discovery
SL. The other thing I noticed is that I saw the press conference by the Royal Astronomical Society and I was wondering why it was it looked so rushed, it was sort of improvised… is there any particular reason for that?
PBR. I would imagine that’s a combination of a couple things. One is we wanted to be careful not to have the story leak. We know how that worked out. But the goal was not have the story leaked and so we didn’t disclose it to even journalists until about a week before, except for a select few [BBC4] Sky at Night got advanced notice and they put together a very professional production. One of our concerns is that we didn’t want to compete with interest with the Mars 2020. The journalists and the sort of the sort of wider media wasn’t informed about this until the week in advance.
The Royal Astronomical Society is a wonderful society for astronomy. I don’t think that they’ve had quite as much experience putting together these kinds of media events especially in light of COVID. I think that’s another reason why this looks the way that it does this would have looked very different, I think, if it hadn’t been for COVID they would all been in the same room there probably would have been more professional equipment to record them. I think that it would look very different if it hadn’t been for the pandemic.
SL. So that’s the illustration I saw from Sara [Seager]’s presentation, where she talks about the various speculations about how life could look like on Venus. I was reading a book by an author, a British author that talks about the danger of presenting this sort of illustrations, because they are kind of deceptive. What do you think? Do you think we should stick to what we know exactly or we should imagine a little bit so that people get inspired? But then of course we know what the dangers are and the same thing happens also with the way that the exoplanets are depicted. I know that those are illustrations, yes, but do the public know about that sometimes not
PBR. There is a risk about this. I’m going to risk contradicting myself. I think in this case actually it’s good to have these artistic representations, partially because I think that it’s important to have this integration of science and art. Art is a very important human expression as is science. I think that there’s something very human about seeing something new and then trying to draw it and even allowing our imaginations into that. I think it’s important to understand that a lot of this will be imaginative, that that this is limited by our own human experience, and that what’s there is probably even stranger more wild than what we could imagine. That’s not always well expressed. I think that certain artistic illustrations are very useful if they show something about the mechanism and in this case I think that the artistic representation is quite useful because it’s showing what would be needed for life to exist in droplets of pure sulphuric acid or 90% sulphuric acid…
SL. And that’s why you need a bubble… a protective bubble.
PBR. Yes… so talking about why life needs this impermeable or near impermeable membrane is one thing. Demonstrating it using sulphuric acid is another, which I think is a useful demonstration. But I think that sometimes people won’t have the right picture in their head unless they see something like this artistic representation, which may not be accurate too. If there’s life there at all does it really look like that? [I don’t know.] But it does represent one of the important mechanisms in a way that can be grasped by the imagination and therefore better understood.
SL. I’ve seen a bunch of videos, where they put the sulphuric acid on sugar yes you see what happens… it becomes carbon immediately… it takes a bit but…
PBR. It takes a little bit of time but you make this sort of worm and then you see the steam coming off and…
SL. What was the sort of review process, how was the paper received by the reviewers? Did you get any nasty reviewers or they were all positive or what happened?
PBR. They were all positive about the implications of this research. They saw that if this was correct, this would have some fairly wide-ranging implications for strange chemistry on Venus. One of the reviewers was Kevin Zahnle. He’s a disclosed reviewer. It’s mentioned in I believe in the acknowledgments. He’s a very well-established photochemist and he’s known to be very sceptical about biosignatures. He’s published quite a bit about why he doesn’t buy methane being on Mars even. He was also sceptical about our results, but he thought that our paper was presented carefully and that it was worth putting out there into the literature so that people could test this and maybe refute it.
SL. Okay, the other thing I wanted to talk about is that there were some antecedents to this paper. So while this paper was being prepared or while you guys were working on it there were a series of papers led by Sara [Seager] and this is one of them, where they hypothesize… they try to think about how life could be on Venus.[6] Was this planned? Did they already know that they were going to publish these [results] and then they were working on this?
PBR. This would be priming the community a little bit.
SL. Preparing them…
PBR. Yeah, well because there are a lot of serious questions about if you want to claim that life is even a possibility there are a lot of things that you want to answer. So you want to prepare the community for what it is that you’re going to be talking about. When this paper was accepted and actually even when it was submitted, the observation on Venus had already been made for quite some time.
SL. Yeah because this is [published in] August and then… yeah… so the second measurement was done in 2019 right yeah…
PBR. Yeah, April or May, I don’t quite recall…
SL. Yeah so let’s talk about you… your website, great website…
PBR. Oh thanks
SL. Do you offer services for websites?
PBR. I can explain how I produced this. HTML5 has a wonderful suite, where you can select different kinds of templates. I chose a template off of HTML5 and it’s very easy to program in HTML if you if you’ve had any programming experience. In fact you can see the HTML5 up at the bottom. You can choose one of the templates. I modified this template somewhat so it looked a little bit more original and then added information.
SL. Is it faster than WordPress?
PBR. I don’t particularly like WordPress, just because I have more trouble formatting it. HTML5 is completely flexible so if I want to spend enough time I can make it look any way that I like, whereas with WordPress it’s very annoying to change it past a certain kind of template.
SL. Okay and so what do you do? What’s your research about?
PBR. So if I look at my website, I’m apparently a naturalist, an astrochemist, and a Cambridge postdoc, and I’m asking the question: are the principles of life origin and evolution universal?
My main interest is in that deep question of how life originated. Could it have only originated one way? Can looking at other planets and even exoplanets help us to test origin scenarios? One of the problems with origins of life is somewhat philosophical and speculative, largely because we have a sample size of one and it’s very hard to test based on a sample size of one.
One of the opportunities that finding life on other planets or exoplanets has is looking at how that life is distributed and perhaps setting up tests for which scenarios are more plausible and which are less plausible. I think the laboratory is another important way to test this more immediately. But it’s a limited test because you might need a lot of time for the chemistry to really work out to make life as we know it. Exoplanets will be laboratories where that time has been taken.
SL. How did you get involved in this work on Venus?
PBR. It’s a really funny story actually I had published the abiogenesis zone paper in 2018 and this is a paper talking about using the light from other stars…[7]
SL. Yes, I love this paper…
PBR. Oh thanks… it’s definitely one of my favourite papers and involves working with some of my some of my favorite scientists…
SL. We spoke about this paper when we did an interview with Professor Didier Queloz. One of the questions was about this paper and I think this work is amazing because what you are doing there is that you’re looking at the effects of UV light in the synthesis of precursors to RNA, right?
PBR. Yes the nucleotides that ultimately would make up RNA. Two out of the four of them can be formed this way. The other two can be formed as deoxyribonucleotides [8] and we will have a paper out soon showing that this works under realistic UV conditions.
SL. In your paper basically you talk about a biogenesis zone yes and habitable zone probably people are familiar with the habitable zone, which is the region in space where we can have liquid water. But what’s this abiogenesis zone?
PBR. That’s the zone where you have enough ultraviolet light from the quiescent or quiet emission of the star. So when the star isn’t being active or flaring there’s enough UV light to drive forward certain prebiotic photochemical reactions that build all of the main categories of life’s building blocks. It builds amino acids, which make up the proteins, which help to catalyse reactions in the cell. It builds phospholipids, which make vesicles which make simple membranes, which can hold the cell together. It also makes these informational polymers or at least it makes the building blocks of those informational polymers, DNA and RNA.
SL. You have a figure in your paper that shows… this one right… which we showed in the interview as well…
PBR. We concentrated on the RNA pyrimidine nucleotides and we specifically concentrated on two of the very early reactions, partially because the later reactions are harder to set up, but they occur over about the same time scale. So the assumption, which we have now actually tested in a forthcoming paper, is that these early reactions will run at about the same rate as later reactions.
Specifically we looked at two sources of electrons. The way that this works is you have particular molecules that end up dissolving in the water and when they dissolve in the water they become anions so they have negative charges and the UV light will knock electrons off of those anions. Those electrons will go and they will attach themselves specifically under hydrogen cyanide and will reduce the hydrogen cyanide and will allow for further chemical reactions that form simple sugars. From there, depending on what else you have around, they either go in the direction of forming the RNA nucleotides or they can go in other directions to form proteins or other directions to form the phospholipids.
SL. How does the setup look like? You have what…? A vial with the some chemical components and then you… what? You increase the temperature or you just put the UV light? What do you do?
PBR. Well the UV light actually ends up increasing the temperature. In this case you’ll notice that it says 254 nm. That’s produced by a low pressure mercury arc lamp. Actually it’s produced by 16 of them, which are arrayed in this cylinder. You put the sample into the cylinder, you turn on the light, you wait a couple hours or, actually in the case of the first reaction, you wait about 30 minutes, and you end up forming this amino methane sulfonate, which goes on to form glycolonitrile. Glycolonitrile will further go on to form glycoaldehyde in the presence of UV light. In this case hydrogen sulphide, we don’t have to use hydrogen sulfide in that step, but we wanted to look at both hydrogen sulfide and bisulfite to see which one worked better. It turns out that bisulfite works much better, this SO32-. It’s more prebiotically plausible and it’s much faster with the chemistry, which is very important. The hydrogen sulfide is too slow even to work on Earth.
SL. So all these things they happen in a relatively simple setup. So you just start from the ingredient, you put a UV light and that’s it and then you wait.
PBR. In this case we do add a couple of extra things. One of the important ones is phosphate. Phosphate is important for later reactions because phosphates are incorporated into the backbone of RNA and DNA but they are also important as pH buffers. They control the acidity of the solution, which helps to control the reaction. They are also chemical buffers. It turns out that there are a lot of side reactions that can happen in the setup. You produce a lot of other products. Phosphates are really amazing for cleaning up those sorts of products and allowing you with very high and selective yields of what you’re actually interested in.
SL. Where do you think we had this kind of conditions?
PBR. That’s a wonderful question and the honest answer is I don’t exactly know. I know of some candidate environments. So the one which was looked at in a lot of the precursor papers that found out about this chemistry, before we actually tested it. This looked at an impactor that would come in. Impacts would generate a lot of hydrogen cyanide and they would expose a lot of iron. The iron would react with the hydrogen cyanide to form these ferrocyanides. So in this impact crater you would form these organometallic complexes and they will complexify with various salts and other things that happen to be around there to form all of the precursors that you need for the sort of chemistry. Then the idea is that you would have it rain afterward. The little streams that would form in different regions do the different chemistries. Then the streams would join and the proteins or the amino acids would meet with the nucleotides and everything would mix and start to interact with each other. There are other scenarios that can do this as well but that I think is one of the best investigated.
SL. We can make the building blocks of RNA, how far are we from getting RNA?
PBR. To get to RNA actually probably not all that far, if you want to talk about short oligomers, say two or three of these building blocks hooked together. It turns out that this chemistry also produces imidazoles, which are these rings that have carbons, nitrogens, oxygens and then various other end members. These particular species actually help to activate these nucleotides and allow them to oligomerize. They allow them to hook up without having enzymes. There’s a very nice paper that’s been accepted in Nature Chemistry, in which Ziwei [Liu],[9] the first author and [also] a lot of the rest of John Sutherland’s group investigate the ways in which that particular molecule can help to activate the amino acids the nucleotides and the phospholipids.
SL. How far away from building a ribosome?
PBR. That is much farther away and that starts to extend beyond John Sutherland’s work and to work by people like Jerry Joyce, Jack Szostak, that sort of work. There’s still a lot of pieces missing. Even once you get there it’s still a huge chasm to getting to even a self-replicating evolving protocell.
The sort of interesting thing about the research is that it’s very much like going on a mountain hike. You think you’ve gotten to the peak but then you find out that the peak is much farther away. The farther we go the larger the landscape becomes and the farther we see we need to go.
SL. There are also people working on alternatives to DNA. I saw some papers from the LMB, from people working on XNA.
PBR. Yes, you are probably talking about Phil Holliger’s group. He’s published quite a bit. He used to work pretty closely with Jerry Joyce and he’s done excellent work in origins, also looking into alternatives to DNA and RNA.
The more you know the more you have your path laid out the more confident you can be about what the best ways are to get where you want to go. But since we haven’t even gotten where we want to go, it’s very open as to which path you should take. My sort of philosophy is I am most confident about a particular path, but this is for largely philosophical reasons or even emotional reasons, not so much for scientific reasons. There’s so much to explore. I might as well stick with my philosophical and emotional reasons and I’ll explore what I explore. Other people with different sort of inclinations will explore what they explore and we should be able to talk with each other and get along with each other and then figure out ways to test which of these are the better ways to go or the worst ways to go once we get far enough.
SL. There are people that think that other life forms - in case there are other life forms outside our planet - would be based on DNA, [while] other people think that they might be based on some other thing like this XNA or something different? Well we don’t know… we cannot possibly know right?
PBR. Yeah and nothing says that it should be just DNA. So there are sort of two conservative kinds of variations that you can do and then there are more progressive sort of variations that you can do. The conservative variations are exactly like this.
XNA means that you’re changing the sugar. So a nucleotide is made up of the phosphate, the sugar, and the nuclear base. You can change the kind of sugar so RNA and DNA depend on these hydroxyl groups at the very end of the bottom of the ribose. But you can change those and one of the ways that you can do this is you end up with a structure like this. And this is RNA. One of the things that you can do is… and I don’t remember which one of these you end up removing… but you can remove one of these and that becomes DNA (deoxyribonucleic acid). Then you have this. Then you have your base here. The two things that you can do is you can change this to a different kind of sugar. The other conservative thing that you can do is you can change this to a different base for DNA… this can also be a T. This is for RNA this is for DNA. There are extra complexities for people who really work in biology. It’s not quite as simple as this, but this is the sort of framework. There are other bases that you can put here including bases that life doesn’t even use. So you can change the sort of end-member groups. This either looks as a single ring, a pyrimidine, or two rings, a purine. You can change the end members or the moieties, the chemical groups at the ends of these sorts of things into things that life doesn’t even use. You can actually come up with alternatives here. You can also come up with alternatives to the sugar. So far nothing works quite as well as what we have. But one of the arguments you could run is maybe, chemically, it’s easier to form something different than this and then by natural selection what we end up with, because it just works better, eventually ends up being selected for. So the sort of chemical changes happen because of a natural selection or just because of randomness. Those are the three sort of options that people seriously pursue is you start with one sort of chemical system and we end up with what we have in modern life either because of just random accident or because of chemical necessity or because of natural selection that it turns out the chemistry favours forming one sort of thing but then natural selection transforms that to something else.
I mentioned that these are the conservative things that you can do. There are more progressive things that you can do. Nick Hud is one person who works on some more progressive ideas about this.[10] Jack Szostak also has where instead of having this sort of system you find other things that bond in entirely different ways.[11] But they can also contain information…
That’s an interesting sound!
SL. I initially had that camera there but then I said if that camera falls down then it’s a problem because it’s gonna break your head. If it breaks my head it’s fine. So I had it there initially.
I’ll tell you something about this paper… no not this one… the previous one we’re talking about. Your paper… I was talking to some collaborators and I told them I would really like to interview the guy that wrote this paper[7] but then I didn’t realize that it was you. I didn’t remember your name and then I found out that then I told you do you wanna do a podcast with me about the Venus paper and then I said “oh that’s the guy that wrote this paper”…
PBR. And this actually does fit into the Venus story amusingly, more into the history of it, I would say that the science in this is not all that relevant to whatever is happening on Venus right now, but I was giving a talk about these results at Imperial College in London and one of the attendees happened to raise his hand. He introduced himself as David Clements - I’d never met him before - and he asked:
“Do you know anything about biosignatures?”
I said “Well not so much I know a little bit”.
He says “Do you know anything about the chemistry of biosignatures?”
I said “Well for some of the biosignatures yes, I do photochemistry, and so I know a bit about them”.
So he said “What about phosphine?”
I said: “I don’t know a lot about phosphine but I know someone who does. [Clara] Sousa-Silva from MIT”.
She was at University College London relatively recently, but she had gone to MIT and she has specifically worked on phosphine as a biosignature, that’s the person you’d want to talk with. She knows the most about the chemistry of it and she knows the most about the spectroscopy of it. He approached me later after the talk and said:
“Well the reason I’m asking about phosphine is we found it on Venus”.
I didn’t believe him. Because I know enough about the photochemistry. I had not really studied phosphine much but I know what phosphine looks like and I know enough about the photochemistry to know that it shouldn’t be on Venus. I kind of assumed Venus would be like Earth. In this case Venus is relatively oxidized. Earth is relatively oxidized. It [phosphine] has a lifetime on Earth that’s so short that it’s all gone within one kilometre height and so it just really shouldn’t be there.
So I dismissed it.
SL. Did they ask you “sign an NDA before I tell you… don’t tell anyone”?
PBR. So actually both Jane Greaves and David Clements were really open about this because no one was really paying attention. Lots of people got to hear about this but no one was taking it seriously. By no one I mean including myself at that stage. But I did say that I would introduce him to Clara [Sousa-Silva] and I think Clara even initially, with the first couple emails, was pretty dismissive. She said “Oh, yeah, I’ll send you some of the stuff that I’m working on and you can look at that”.
Then I got back to Cambridge and it turns out Jane Greaves had a visiting professorship at the Institute of Astronomy in Cambridge. So we ran into each other and she had been included in some of these emails. She sat down with me and showed me the data that she had from the James Clerk Maxwell Telescope and when I saw the data I got more excited because this looked like a pretty good detection. Jane’s a very careful scientist and so if she was confident, I was more confident. We got more excited. We started talking so loudly in the common room in fact that a few of the members of Cavendish Labs got to learn early about the detection of phosphine on Venus.
What I did was I emailed Clara back with some of those results with Jane cc’d. Clara got a lot more excited and involved Sara Seager. Sara Seager proposed submitting for time with ALMA (Atacama Large Millimeter Array). Jane said “Well I’ve already done that a few times and I keep getting rejected”.
But we were pretty sure we could send a much better application now for two reasons. One is we had a theoretical basis. We started looking at the stability of phosphine on Venus. It’s much more stable on Venus than on Earth because on Earth you have a lot of water vapour and water vapour makes a lot of hydroxyl radicals, which are very efficient at destroying phosphine. It’s one of the reasons why you tend to have these parts per trillion amounts that end up getting completely annihilated within a kilometre. Whereas on Venus these part per billion amounts, which were being suggested by some of the results by the JCMT (James Clerk Maxwell Telescope) measurement, made sense. So we could argue that it made sense.
Additionally Jane had her data now and could show that to ALMA. We got a response very quickly within a couple weeks, which is normally you have to wait months for these applications but we got a response a couple weeks saying I think we can see Venus within a couple weeks and they did. They got the data. The data was very difficult to reduce. So it took months before we finally knew that phosphine had been seen with ALMA.
When that was known I went out for drinks with my wife and I can barely remember even where we went or what we talked about because it just felt otherworldly.
SL. And then what happened?
PBR. So once we saw it that’s when the astrobiologists really went to work. Janusz Petkowski and William Bains, both of them are top-notch astrobiologists, had done some work on the astrobiology connected with phosphine, and along with Sukrit Ranjan and myself, both of us are photochemists, we really started to look at the alternative ways to produce phosphine on Venus. We looked at a lot of alternatives. It ended up becoming a 100 page paper[12] that we attempted to get accepted at a certain journal. I won’t name the journal, but the journal rejected it because it was too long.
SL. 100 pages?
PBR. Yes, and that 100 page paper is accessible you can get it off of arXiv. It basically goes through all the ways that we looked into forming this. It turns out that any known abiotic production that we could find in the literature anywhere was not sufficient to form the amount of phosphine that was observed.
SL. So basically these results are just mentioned… simply mentioned in this graph right, which is from extended figure 10. So basically on Earth we have two ways of producing phosphine right so one is the industrial and the other one is from microorganisms…
PBR. Microbes in anoxic environments. I won’t get much more specific for two reasons. One is that I am not a biologist, I don’t have a biology background. The other reason is I’ve talked with the biologists and they don’t know. So they think that it’s a particular E. coli. But the mechanism is not known. Even the particular species is not known, according to them.
SL. One thing I was curious about… so we know that maybe there is some bacteria that produces this phosphine, but what are the surrounding conditions that we need in order… so what are the starting ingredients in order to produce phosphine? What do you need?
PBR. Hydrogen and phosphorous. Life is actually very good it almost looks like a sort of LEGO set and again we don’t know the mechanism, but if it’s like a lot of other enzymes then what it probably does is it assembles things together like hands assemble LEGOs.
This is very much the way in which for example adenosine triphosphate is produced in a cell. You have adenosine diphosphate. That last phosphorous bond is very energetic it’s where a lot of energy is held within cells and is produced by ATP phosphatase, which basically just has this little rotor that has a proton gradient and that moves a little rotor and the rudder just snaps the molecules into place.
I would imagine that phosphine is being produced in a very similar sort of way. It’s really amazing how life works mechanistically to form molecules. It’s almost the way in which you imagine molecules to form when you’re a kid. You have these ball-and-stick models, you just click them together. Life clicks them together.
It’s different from abiotic processes where there are these more comprehensive sort of reactions, where unnecessary bonds get broken and reform. You have all these intermediates. It’s a very different sort of system.
SL. So what do we have here, we have a different production pathways of phosphine… I read that you can produce phosphine also within volcanoes…
PBR. Yes in principle. In practice not on Earth. Not in any known volcanoes on Earth. It might be interesting… this is a bit speculative… but it might be interesting to look on mud volcanoes like in Azerbaijan. Those mud volcanoes produce a lot of methane and so they’re very reduced. The reason that they end up producing the methane is probably because there’s a lot of decaying life that gets reprocessed and turned into the methane. But in most volcanoes the constituents are carbon dioxide and water with some sulphur dioxide and sometimes elemental sulphur, and hydrogen sulphide is about as reduced as you get. You need to become much more reduced than that to start producing phosphine.
Also our geology is fairly phosphorous limited. So there’s just not as much phosphorus to work with, to begin with. If you had a much more phosphorous rich mantle that was degassing it would have to be more reduced than as reduced as we imagined most of the early Earth magmas to be, by about seven orders of magnitude. At that stage you could start to really produce a lot of phosphine. There’s some pretty good evidence from what little we know about the mineralogy on the surface of Venus that it is not nearly sufficiently reduced.
SL. What are the other production mechanisms you talk about… you hypothesized?
PBR. So near the surface of Venus is very hot. So one of the things you could imagine is maybe there’s an equilibrium thermochemistry. So it’s hot enough to just break bonds and reform them. So you can imagine kind of shuffling the atoms around and maybe some of those atoms end up looking like phosphine. This is in fact how phosphine is produced on Jupiter and Saturn. It’s a very abundant constituent, but it’s very much like shuffling cards. If you have a whole bunch of hydrogen cards around and your phosphorus card and you shuffle it, it’s very likely that your phosphorous card will be next to the hydrogen cards and so you end up forming phosphine.
On Venus you have a lot more oxygen and not very much hydrogen and so when you shuffle these sort of atomic cards, you end up with something that looks more like phosphates or various other species with phosphorus bound to a bunch of oxygens. So that’s what we find from the thermochemistry. The amount of phosphine that’s produced is very tiny.
This is also the problem with lightning. Lightning is kind of like thermochemistry but just much that’s another way. It might produce some phosphine. It produces very little because it’s done with the same mechanism, but just at higher temperatures and then…
SL. And this lightning where does it happen in the Venus atmosphere?
PBR. That’s another great question. So little is known about um about Venus. There are some radio observations. I’m not very familiar with them but there are some radio observations that are indicative of discharge processes. So there is indirect evidence of lightning on Venus. No one’s seen any optical evidence for lightning on Venus yet.
SL. What would you use to detect the lightning in another planet… well I mean you can use a light sensor because it emits in the visible…
PBR. That’s the best way to do it… there you there you go… and that’s the way that it’s seen on Jupiter and Saturn. There are these beautiful images of lightning strikes they happen within the water clouds of Jupiter and Saturn. If you get deep enough there are these water clouds. It’s gorgeous there are these enormous storms. The size of the lightning is much greater than the size of lightning on Earth.
In Venus, maybe there’s storms that happen. They just happen deeper in and so they’re a little harder to see. There might be more small scale or there may not be these kinds of storms that produce lightning at all. There’s a bit of a chemical signature for it as well. There are some of these NOx’s [nitrogen oxide] that have been seen in Venus’s atmosphere. The best explanation for the existence of these NOx’s is that the nitrogen, which is the second most abundant species in Venus’s atmosphere was dissociated. The only real way to dissociate that, at least deep within the atmosphere, is with lightning. You need a lot of energy to break that triple bond. Then it might as well bond with oxygen. So you end up forming NO and O2.
SL. So we didn’t see any lightning with the probes we sent to Venus?
PBR. No, no, no…
SL. You guys said also asteroid impact could be another option…
PBR. Yeah so this can produce phosphine in two different ways. One is it delivers reduced phosphorus species. There’s this mineral called Schreibersite, which is a reduced phosphorus material. What we did was we calculated how much phosphorous would be delivered by just the interplanetary dust impinging on Venus and we said “Let’s just imagine that every single atom of phosphorus was converted into phosphine”. That was ten thousand times too little to explain the observations.
The other way in which you can produce it is with a much larger impact, which has two effects. One is it does deliver some phosphorus, but the other thing is that it delivers a lot of iron. The iron will reduce the phosphorus that’s already present there. This actually could produce enough phosphine to explain the observations, but it would either be such a large impactor that surface features would be seen. There has been surface map mapping there are these infrared windows, where you can see to the surface and we should really see a crater there and additionally these events are so rare that you would only expect them happening once every million years or less and the lifetime of phosphine is on the order of less than a thousand years, more likely around 100 years. So you would have to have had that sort of event in the past 100 years probably and that’s unlikely.
SL. This calculation of the phosphine lifetime is something you’ve done with your modelling, if I understood well, in this graph. So we spoke about ways of producing phosphine. We didn’t talk about the life hypothesis yet. I mean, we sort of discussed that, but I wanted to know more about this graph. Can you explain me this thing? What was that?
PBR. Yeah so this is the output of a photochemical model. [And this] photochemical model includes um hundreds of species thousands of reactions which can be written out as these differential equations, which are solved numerically…
SL. Do you want to explain what’s a differential equation to the YouTube public?
PBR. Yes so, in this context, I think it’s best seen as rates. So there’s a sort of rate at which something is forming or is destroyed and this is written as a combination of the abundances of the reactants multiplied by a rate constant, which is how fast these reactants work together to form the products and that equals the change in the amount of products over time. That’s the differential part of the differential equation. It’s talking about a change in the amount of something over time.
SL. So what did you get from this model?
PBR. Yeah so this model got a bunch of different things. The sort of precursor is a slightly different picture than this one which is typically called a spaghetti plot and this shows all of the major constituents that have been observationally constrained within the atmosphere of Venus or all of the relevant ones that we could model with this particular model…
SL. Is it the one in the supporting information?
PBR. Yes it’s there this one. That’s the one.
SL. that looks complicated.
PBR. Well it’s complicated because there are a lot of different molecules but what this is showing is that the changes in molecules as a function of atmospheric height predicted by the model match what we know. So this gives you some confidence about that the model is reproducing. What’s happening in Venus’s atmosphere at least reasonably accurately. It doesn’t match everything, it doesn’t do very well for water in the upper atmosphere, for example.
SL. What’s the mixing ratio?
PBR. So a mixing ratio is if you take all of the molecules per cubic centimetre of a particular species, say SO2, sulphur dioxide, and then you divide that by all of the molecules in that cubic centimetre, that gives you what’s called a volume mixing ratio or mixing ratio in this case for short.
It’s a way to talk about the fraction of the atmosphere that is whatever it is.
SL. So basically what did you conclude from this graph?
PBR. What can we conclude from this? Uh from this graph, from this graph so this is something that was taken from the results of that model and what this is figuring out is this is figuring out based on minor constituents in the atmosphere as well as based on the temperature and pressure and what we know about how phosphine reacts it figures out how long phosphine should be able to survive in that atmosphere, that’s the blue line.
The red line is a more hypothetical graph. That’s plotting the formation based on just photochemistry. So there’s a little bit of photochemistry that can form the phosphine the rates at which this is done are fairly uncertain they’re very uncertain especially at the last stages but ultimately it takes something like PO2, it reacts that with hydrogen to form hydrogen bonded with PO2, the oxygen is eventually abstracted away, so you get something like HPO and eventually you get HP and then H2P and H3P. There are a variety of pathways to that. There’s a wonderful picture showing all of these different pathways. That’s how much it gets in terms of this is the rate of formation with the most favourable estimates including the estimates of the unknown reactions. Then that’s compared with the destruction.
The formation and destruction can be balanced in two ways the destruction rate that’s shown in this picture is the destruction rate that’s implied by the observations so the more that you have of something the faster it’s going to be destroyed and that’s why formation and destruction look so different.
If you make the formation and destruction fit in this particular plot, then you end up with something like the dotted line in the next picture which shows the mixing ratio and that dotted line shows how much you would expect with just the photochemistry, which as you can see is many orders of magnitude. This is a logarithmic plot. So it’s many thousands of times lower than the amount that has been observed.
So that’s how you end up reading this.
This also importantly says how long you have to wait until your phosphine goes away. So if you just had say a source from an impact or something how long do you have to wait until it goes away well you just take 10-10 seconds to the -1, you invert that, and that works out to 100s of years.
SL. So I’m interested in this in this graph… so you plot the destruction at a certain altitude, how long it takes for phosphine to be destroyed, and now when you are on the surface it’s going to be fast because it’s very hot… I mean… is phosphine flammable?
PBR. It’s flammable on Earth because there’s oxygen available. It is still destroyed at high temperatures near the surface of Venus and is destroyed by thermal dissociation. It just hits a third body hard enough that knocks off one of the hydrogens and is unlikely to find that hydrogen ever again.
SL. Then what happens here? I’m very curious about this feature here… what happens around the 20-30 kilometres…
PBR. So what happens there is… at that particular atmospheric height, that’s right below the clouds. So as the sulphuric acid from the clouds comes down it ends up evaporating it goes back into the vapour and then it ends up dissociating into SO3 and H2O. Then the SO3 ends up reacting with some of the sulphur that’s available from the OCS. There’s a whole series of reactions that happen and that generates a lot of extra oxygen atoms, hydroxyl radicals, because you’re introducing water. It also generates, through reactions with a hydrochloric acid, that’s also present in Venus’s atmosphere, it generates some free chlorine atoms. All of those end up destroying phosphine and so when you get to the bottom of that cloud layer it turns out that you are eating away a lot of that phosphine through the reactions with radicals.
The dotted line ignores the amounts of radicals because the amounts of radicals are usually on the order of say a thousand molecules per cubic centimetre, which if that’s all that was there would be better than most laboratory vacuums. It’s a very small constituent and is very uncertain. Every model gets a very different answer for that sort of curve. So we included the dotted line curve and we actually based our results on that dotted line curve because we didn’t want the results to be too model dependent. It’s more conservative to say it can only be destroyed by thermal dissociation and by photodissociation, which are fairly well determined. That’s what causes the dotted line to raise up.
SL. So when you get there, there are less clouds. So you get more UV light, correct?
PBR. And then the reason for the time scale in between those is because of diffusion. It would actually live a lot longer if the atmosphere was not moving. You could actually have it survive for thousands of years, if the atmosphere didn’t move. But because the atmosphere is constantly circulating, the phosphine gets circulated into regions where it does get destroyed and so its lifetime is determined by its diffusion through the atmosphere. This is what’s called Eddy diffusion, which often times is driven more by turbulent motions. So the small-scale motions in there. It’s very slow in Venus’s lower atmosphere, it’s exceedingly slow, two orders of magnitude slower than on Earth, except in our cold-trap. We have one small region in our atmosphere that’s very slow as well.
So this is longer. This is shorter. This up here is shorter because of the UV light directly destroying phosphine. The reason why this breaks off as well is the same reason why it breaks off here. So at the bottom of the clouds you get these radicals and atoms. At the tops of the clouds, the photodissociation doesn’t just photo dissociate phosphine, it also photodissociates SO2 to form SO and O and that O goes on and destroys all of your phosphene.
SL. Okay, your model basically is based on what we know about Venus, but what do we actually know about Venus. I mean do we know everything we need to know or there are so many parameters we don’t know, and those are just a guess work. How many of these parameters that you put in your model are guessed?
PBR. A lot of them. I don’t know the fraction. For Venus it’s very difficult because there’s a lot of important reactions that involve sulphur and chlorine and both sulphur and chlorine. Chlorine chemistry is difficult to study, especially with hydrochloric acids and things. Sulphur chemistry is exceedingly difficult to study, because sulphur reacts very differently from oxygen. Oftentimes it’s seen as though oxygen is a sort of analogue to sulphur and a lot of reactions are built by just looking at say CO2 reacts a certain way, various other species may react a different sort of way. So you look at CS2, for example, as an analogue for CO2. That’s not usually very accurate. A lot of laboratory work needs to be done with sulphur chemistry. It’s exceedingly difficult because sulphur is a lot more prolific in its reactions than oxygen. Oxygen likes staying at this state of minus two. It likes forming these two bonds. Sulphur can end up forming two bonds or four or six. There’s a lot of different things that sulphur can end up doing.
There’s an incredible amount of uncertainty even in what’s in the atmosphere of Venus because it’s hard to see below the clouds. It’s difficult to know what the droplets are in fact made of. There’s no real good measurements of that. VEGA was able to see a little bit of what the clouds were made of, possibly in one region. It’s unlikely that the clouds are completely homogeneous. The reason why we think they’re primarily composed of sulphuric acid is entirely due to models with these uncertainties. We need to start measuring things in the atmosphere. We also need to start measuring things in the lab to really better understand what sort of chemistry can happen in these sorts of environments and what we should expect.
SL. So why we cannot do spectroscopy to understand what’s in the atmosphere? Where is the difficulty?
PBR. Well the difficulty is you can only see so far. So even with the phosphine it turns out that there’s a continuum due to the clouds beyond which you can’t see any deeper. So the line against the continuum limits you to seeing only above [about] 60 km. The upper atmosphere of Venus is much better understood than the middle atmosphere and the lower atmosphere for this very reason. There are certain atmospheric windows, which allow you to see deeper and that’s been done for water and for sulphuric acid and for sulphur dioxide. There’s been some excellent work looking into the profiles of these species and coming up with what their concentration should be as deep as 35 km from the surface or even 25 km from the surface. They’re still somewhat limited. They’re often highly uncertain. They give us some very good constraints about how much of those species are below the clouds. Those can only be done for a handful of species and usually only the most abundant ones.
SL. So for sure we know way more about Mars than Venus…
PBR. Oh, yeah. The fact that you can have a lander go there and that the lander isn’t destroyed in a matter of days if you’re lucky… Although now there are ways to build landers that could survive for potentially a month or more on Venus… but still that’s a very short amount of time compared to how long you can have lander going around the surface of Mars. Martian atmosphere [and] climate history is much better understood. Mars is also better understood just because the surface is much older. Venus’s surface… there’s some evidence that Venus has been completely resurfaced in the last 600 million years or so.[13] So we’re very limited even if we did send a lander there we would learn what Venus was like recently. We might learn about why it ended up like it was but we wouldn’t know much about what it was like before.
SL. Building a rover for Venus would be extremely difficult because I mean you need to have a way to cool it down, right?
PBR. Yes, you basically have to send a refrigerator and you have to keep it cool enough so the instruments can work long enough to get results…
SL. And solar panels there are not gonna work well because it’s very cloudy, right?
PBR. Yeah, there might be alternative ways to get energy, but those would be very difficult with standard rover technologies. At the same time, I think the real limiting thing is you’re near a surface which can melt lead and which destroys most electronics within a matter of minutes to hours if they’re not shielded. Even if they are well shielded [the electronics will break down] in a matter of days.
With future rover concepts you might be able to get things that last for a month or more, which would be a considerable amount of time and you’d learn so many interesting things from the surface. But it is hard to sell that to a funding agency saying you can have a mission that lasts for a few years or a mission that lasts for a month if you’re lucky.
SL. Now the thing I was curious about, is that the most important graph which is this one… the most important graph shown in the in the paper is this one or the other one I can’t remember, which one is it, which is the one where you it’s basically a spectroscopic graph, where you show an absorption line from phosphine that was measured with those two telescopes. The thing I was curious about is that what’s the excitation source for these measurement and where does it come from?
PBR. All right so this is going to start also getting outside of my area of expertise pretty quickly because I am not the observer. So I know that this is the zero to one transition it’s a rovibrational mode of the phosphine and the way in which it actually gets excited it starts in its ground state so it starts in the ‘0’ state and it ends up getting knocked into the ‘1’ state both from light from Venus itself and then also from reflected light from the sun. Goth of those contribute a certain percentage. When people look, they notice there’s a little bit of light missing around one millimetre, it’s an absorption feature that’s the reason it points down. So you aren’t seeing the emission from a from excited phosphine; you are seeing the absorption of a certain fraction of solar light and Venusian light. I don’t know what the fractions are because I wasn’t the observer. You are seeing the absorption feature as the phosphine is knocked from that zero state into the one state.
SL. Are we sure that this measurement is correct and this is not an artifact? What did you do to check that…?
PBR. You are looking at the best alternative candidate right now. That’s actually what this plot is showing. There is an SO2 feature that that would contribute to that line. It turns out it’s an excited SO2 feature. It’s very difficult to get it to be deep enough and it’s not quite at the centre either. It’s a little off-centre. You need to be at a relatively high temperature to get the SO2 into the excited state so that it can get into a different excited state by another absorption. The only way to do that [iss to populate the line. The line] tends only to be well populated around 600 degrees kelvin, 600 K, and the clouds of Venus are much colder than that. So it’s not a very well populated line. That’s the best candidate. Clara Sousa-Silva and Hideo Sagawa looked at hundreds of possible candidates and modelled them. This was the best one.
SL. What about Clara’s paper about phosphine as a biosignature[14]… that was very interesting it came out… when was that? It came out in January… this is a very important paper because it suggests that phosphine can be considered as a biosignature…
PBR. It’s a very powerful biosignature because in a lot of contexts it really has no good alternative explanation…
SL. Your paper[1] refers to this paper[14]…
PBR. Of course… in a sense you can see this as a sort of dialogue between two scientists: Clara Sousa-Silva and Jane Greaves. Although they came upon this independently. Clara Sousa-Silva while working as part of Sara Seager’s group looking into phosphine as a biosignature and then Jane Greaves finding an old paper by Glindemann pointing out that phosphine is produced by life and wondering if it might be on Venus.[15]
What this paper does is it really establishes a prediction for Venus, if Venus had the microbes. It turns out that that prediction is pretty well matched by the observations. That of course doesn’t show anything in terms of whether there’s actually life there, but it does show that if there was life there and it was a sort of phosphine producing life, then the signal that we see is what we would expect according to this paper. So if it is, say 20, 30, 40 years from now, established that there are microbes in the clouds of Venus, this will be really important because it’s a really nice prediction before you saw it, then you see it, then you see the evidence for it. It has huge implications for exoplanets as well. It gives a lot of confidence into biosignature claims.
SL. So what this paper[1] is saying is that we found phosphine, we don’t know how this phosphine can be made without processing processes that involve life yeah so it could be life…
PBR. But it could also be unknown chemistry just as I’ve been talking there’s so much that’s not known. I will emphasize again the best way to find out in the short term is by laboratory work and by certain ground-based observations in tandem. You can’t do one without the other to really learn what’s there.
SL. And so in a lab you would try to produce phosphine using different methods…
PBR. So the way that I would do it in the lab is I would start with some droplets of concentrated sulphuric acid with some water in an anoxic environment with a lot of CO2 and SO2 around. I might even enhance the amount of SO2 to see some chemistry a little faster. Then I would add some phosphates in there, which would pretty quickly become phosphoric acid. I would see whether I could get a phosphorus acid, which can disproportionate to form phosphine. I would see how this might work with the presence in the absence of UV light. I would see how this might happen with the presence of a sort of electron gun. I would actually see what would happen if I added salts or various other sort of metallic components into the droplets because we don’t really know what the droplets are made out of. So I would just play around with that sort of chemistry.
The other thing that you can do is you can explore acidophiles. They can’t quite survive in concentrated sulphuric acid but you can still try them in fairly acidic environments and see: Are they more effective at producing phosphine or less effective at producing phosphine?
These are the sorts of directions that I would go with next in terms of investigating this in the lab.
Then the other thing that I would do is I would figure out what other things are being produced. Could they be seen spectroscopically? What does the spectroscopy really look like? I would use those to inform the ground-based observations and make predictions for future observations.
SL. Is there any plan to send probes to Venus now? Have things changed after this paper?
PBR. That remains to be seen. I hope that things have changed. There are two proposed Venus missions through ESA (European Space Agency) right now. There are proposed missions that involve Venus. One involves putting a lander on the surface of Venus. I think that those missions hopefully will have a bit of a better chance because of this there are no balloon missions currently under consideration. There are some missions considered by NASA. I think that there’s a lot of impetus as long as these results hold together. There’s a lot of impetus to actually send missions like this.
SL. These make sense because they’re going to last longer… right?
PBR. Oh absolutely, they will struggle a little bit in sulphuric acid clouds um but they will last considerably longer. They don’t get to see the surface, which is disappointing. But they would be able to look into the clouds, maybe even sample the clouds, figure out what the clouds are made of, and even figure out maybe where the phosphine is coming from. But they can’t do that realistically without the lab work and the observational work, because where do you look? The clouds are big. Do you look at a particular height? What do you look for? How many droplets do you need to collect? How do you collect them?
There are a lot of unknown technological developments that need to be made and they need to be directed by what the laboratory work and what observations say is the best place to look to answer this and other important questions about the constitution of Venus’s clouds. There have been proposed missions like this in the past they’ve always been rejected…
SL. These sort of balloons?
PBR. Yeah, I think that this is a good impetus for proposing a mission like this in the future.
SL. Do you think things are going to improve in terms of interdisciplinary research funding now after this paper?
PBR. Also very hard to say but I’m hopeful that it will. Interdisciplinary work is exceedingly important. I mean this paper really shows how successful interdisciplinary work can be. I’m funded by the Simon’s Collaboration for the Origins of Life (SCOL) by the Simon’s Foundation.[16] That collaboration is a wonderful interdisciplinary collaboration that involves molecular biologists, cell biologists, biochemists, organic chemists, geologists, astronomers, and atmospheric chemists. All of these people work together to try to answer a common question. This paper is a sort of microcosm of that. In that you have astronomers, atmospheric chemists, astrobiologists, all working together. This is a very small subset. There are a lot of other people, including a lot of people who’ve worked on phosphorus for a long time and a lot of people worked on Venus a lot longer than we have that can now weigh in on this, but only if they can receive the funding needed to do the experiments and the observations.
SL. Because Professor Didier Queloz said that it’s very hard to get funding because let’s say that you’re doing a collaboration between two fields you go and ask the founder for one field and they tell you go ask [founders from the] other field so… the Simon’s Foundation is a private philanthropy institute…
PBR. Yes, so it’s funded by contributions by Jim Simons. Jim Simons is a mathematician. He’s famous for the Chern-Simons theory, which is connected to string theory in ways I don’t understand. After he did this sort of work in pure mathematics, he got interested in how to predict stock market and it turns out he was very good at it and became a billionaire. He started funding lots of things including paying for people to teach mathematics to high schoolers, autism research, pure mathematics research, a lot of machine learning. He has various collaborations including this collaboration on origins of life.
SL. There is also another one, the Breakthrough Initiatives as well. I heard that they are interested in putting some money for the Venus… some Venus mission… sending a probe there.
PBR. Yeah, I’m not exactly sure what the money will be used for. I’m hopeful that some of the money will be used for the laboratory work that the Seager group is capable of doing… I think that that’s the most important first step…
SL. So these are the guys that want to send a probe to Alpha Centauri, right?
PBR. Yeah so this is Yuri Milner. I think that this is a wonderful blue skies sort of project to work on because even if it fails you’re going to learn so much about future technology with it. It’s important, I think, to have a broad vision and look toward the stars. This is a perfect sort of thing to do that. I think that there are some great things also to do a little closer to home.
SL. Solar flares. So we discussed solar flares and… we spoke about solar flares… you said surface in certain a certain in certain planets orbiting certain types of stars might be a condition necessary for the emergence of life… but when do they become dangerous and is it a problem for us?
PBR. For us is a very different question than for starting life. The real problem that they pose for starting life is more on these low-mass stars, these ultra-cool stars like TRAPPIST, which has seven planets, three possibly within the habitable zone. It’s much closer to its host star and so it’s much more affected by these flares and the stars tend to be exceedingly active. The energy of the flares is much higher compared to the quiescent energy, the energy when it’s quiet compared to the sun, which is a relatively quiet star. This is really good potentially. Especially when they’re young, when they’re young they’re more active. Just like us… This is really good for starting life if you have an atmosphere. But it’s [also] really good for removing an atmosphere. So it’s still an open question as to whether there’s a nice window, where it flares enough so that you can do this amazing chemistry, but it doesn’t flare so much that it strips you of the atmosphere or it can also just strip the atmosphere of its hydrogen, which is a big problem because then you actually end up stripping all of your oceans and you are left with an O2 rich atmosphere. Oxygen is wonderful for us, but it’s terrible for prebiotic chemistry and is poisonous for almost all early life.
SL. We had the bad flares, solar flares in the past. One of them was the Carrington Event [in 1859]… which burned all the telegraphs…
PBR. I heard that you could actually work with some of the telegraphs days after… they would still function off of the electric energy that you didn’t need to supply them with any energy. They just had that energy contained. There is some projections that if something like that happened now it would destroy many of our satellites and it would throw us almost into a sort of temporary pre-industrial age. I don’t know exactly how realistic that is but it is something to consider.
It’s nice to compare with these ultra-cool stars. Some of these ultra-cool stars, even the quiet ones, will have Carrington-level events multiple times a week.
SL. Are you prepping yourself? Are you a prepper? You know the guys…
PBR. Oh yes… so… no. I have enough food for to feed our family for a couple months and enough water to keep us well hydrated for a couple months, probably not so essential for Cambridge, but still that’s the sort of store that we have, if things get serious beyond that, then I’m screwed.
SL. If you wanna prepare yourself for a solar flare you would need a Faraday cage basically…
PBR. Yeah so if you wanted to protect your own electronics although that’s fairly ineffective because everything’s networked right now if you didn’t have the internet how useful is your computer really…
SL. You connect with the other preppers…
PBR. Yes you make a new internet, we could make a dark web that was specially shielded, we could start launching our own satellites through a private company with Faraday cages around them and see how that works…
SL. Do you look into alien stories… are you into these things?
PBR. I’m not very into these things. I do happen to know a little bit about Ancient Aliens because it was on Netflix and my son Henry is really interested in ancient Egypt and he found out “oh this is a documentary about ancient Egypt”. He started watching it and pretty quickly we found out that this is not what I would call a documentary about ancient Egypt. It’s very imaginative though. But the thing that was most shocking to me, which brings us almost full circle is that one of the people that they interviewed on this documentary for Ancient Aliens, which included the meme that you showed. What’s his name…Giorgio Tsoukalos. One of the other people that they interviewed was a young Sara Seager. She appears in those documentaries. She says very sensible things. Then they interview someone else, who takes those sensible things and mangles them. So I was very surprised to see her in those documentaries, when I was watching them with my son. Because of that actually I did end up watching all the documentaries, not with my son. I didn’t want him to…I didn’t want his head to be to be filled with so much nonsense.
SL. It’s entertaining. I mean there’s a lot of science fiction, but…
PBR. It’s very imaginative. I think this is the sort of thing that all scientists have to balance. It’s good to have a very active imagination, but it’s also good to be sceptical. There are some scientists that are too imaginative and they end up going off the deep end and they end up believing things that they really don’t have sufficient evidence for. But there’s also the risk that you become too sceptical.
SL. You dismiss everything and then you can’t possibly do anything. Even doubt about yourself …
PBR. You can start to become a solipsist. Actually I think that you see both of these in conspiracy theories. You’ll see conspiracy theorists believe all these wild things, but then you’ll see other conspiracy theorists who are sceptical about whether the Earth is flat or round because it looks flat to them outside their window. But they don’t accept any of the other evidence.
Some of them are extreme believers and very imaginative and some of them are just extreme sceptics with no imagination at all. They get to a very similar place.
SL. I had a chat with the Michael Shermer who is a sceptic and the president of the Skeptics Society.
PBR. Yeah, I used to read his articles when I got Scientific American. So, back when I was in high school…
SL. He published a huge amount of articles. These are his books… this is his latest book.
PBR. I used to read his book “Why people believe strange things” when I was in high school. That was a really influential book and helping me to think about extraordinary claims.
SL. So I want to show you the video… so basically these videos were released by… they were released by a guy that used to work in some government agency called Luis Elizondo. One of them was taken in 2004 and the other two in 2014-2015. They show something weird… oh sorry…
PBR. I’ll put this back on. Okay. That’s better.
SL. So that was recorded from a fighter jet. Anyway there are another two videos. Basically so we spoke about these things with Michael Shermer. These videos are been confirmed… that they are original… by the government.
PBR. I’ll trust you about that…
SL. But they don’t know what it is… but then people say well these are aliens…
PBR. Okay so I’d be interested in what Michael has to say about that. My own attitude is we had comparatively a fairly mundane discovery in our paper. We discovered something really neat but it’s not intelligent life visiting Earth. We tried to keep this a secret for one week and failed utterly. It’s very hard for me to imagine that the government, just human beings in general, would be so efficient at keeping a secret. I also, in a sense, I would say that I respect conspiracy theory when there’s evidence for conspiracy theory. I could kind of imagine that the government might do things like this because they are in fact working at say a nice stealth craft… someone got a good picture of the stealth craft… they don’t want to admit that they’re working on the stealth craft… and so they share this stuff they make it sound mysterious…
SL. They are gonna tell you “these are aliens, these are alien spacecraft”.
PBR. They won’t even [need to] say that it’s aliens. They just say “look at this weird thing” and they know what people will do with it. Then they don’t comment on it further. There’s this huge disinformation. I mean Russia does the same thing with people’s political stuff on the internet. This is the sort of conspiracy that I would buy a lot more than intelligent alien life visiting Earth.
SL. We discussed this thing with Michael. In fact there were there were guys tasked to feed the false information to UFO researchers and UFO believers…
PBR. Well then they’re partially to blame for what we’ve ended up with. That doesn’t surprise me nearly as much as intelligent alien life would.
SL. Okay Paul thank you very much and it’s been a pleasure hopefully we can do this again another time maybe with some new discovery hopefully.
PBR. Absolutely it’d be a delight to talk with you again at some point time in the future.